Ecotope
Experimentation platform for the construction of large ecosystemsclient: self
my role/scope: original idea, design, writing
type: freelance
We have spent the last 60 years of spaceflight advancing technologies that help us monitor, manage and predict planetary activities and phenomena. Today the major Earth research organizations of the world are Space agencies, studying ecosystems with powerful observation and predictive models from a great distance. This distance, however, means we are no closer to the crucial milestone required for repairing Earth’s compromised biosphere and settling Space: being able to reproduce an ecosystem outside of its natural occurrence.
Reproducing functional, large mass ecosystems is proving to be elusive. Prior efforts based on notions of replicating Earth have failed and even recently announced proposals seem to want to go in this direction again. These heroic projects, with their large-span transparent enclosures, capture the public imagination. Perhaps they remind us of what the future was supposed to look like. Perhaps we need new imagery. Or perhaps we need a vision that isn’t a thermodynamic fiction.
Ecotope challenges the paradigm of monolithic artificial ecologies and proposes instead a unitized approach that grants control over connectivity, the essence of all networks.
Ecotope would avoid the lose-lose fates of single-volume ecosystems which either fail, collapsing funding and public support and possibly endangering lives or succeed but are irreproducible
Researchers will treat individual modules – each hosting a biological community – as building blocks of landscapes. These arrays permit physical exchanges such as the passage of chemicals and colonizing organisms between modules; connections that operate like corridors found in natural ecological landscapes. Since complex networks like ecosystems are largley defined by connectivity, without a way to control connectivity, design with not be possible. This is the central thesis of Ecotope.
The aim is to finally allow researchers to design systems that work, without getting snagged in Earth-replication games.
![]()
Reproducing functional, large mass ecosystems is proving to be elusive. Prior efforts based on notions of replicating Earth have failed and even recently announced proposals seem to want to go in this direction again. These heroic projects, with their large-span transparent enclosures, capture the public imagination. Perhaps they remind us of what the future was supposed to look like. Perhaps we need new imagery. Or perhaps we need a vision that isn’t a thermodynamic fiction.
Ecotope challenges the paradigm of monolithic artificial ecologies and proposes instead a unitized approach that grants control over connectivity, the essence of all networks.
Ecotope would avoid the lose-lose fates of single-volume ecosystems which either fail, collapsing funding and public support and possibly endangering lives or succeed but are irreproducible
Researchers will treat individual modules – each hosting a biological community – as building blocks of landscapes. These arrays permit physical exchanges such as the passage of chemicals and colonizing organisms between modules; connections that operate like corridors found in natural ecological landscapes. Since complex networks like ecosystems are largley defined by connectivity, without a way to control connectivity, design with not be possible. This is the central thesis of Ecotope.
The aim is to finally allow researchers to design systems that work, without getting snagged in Earth-replication games.
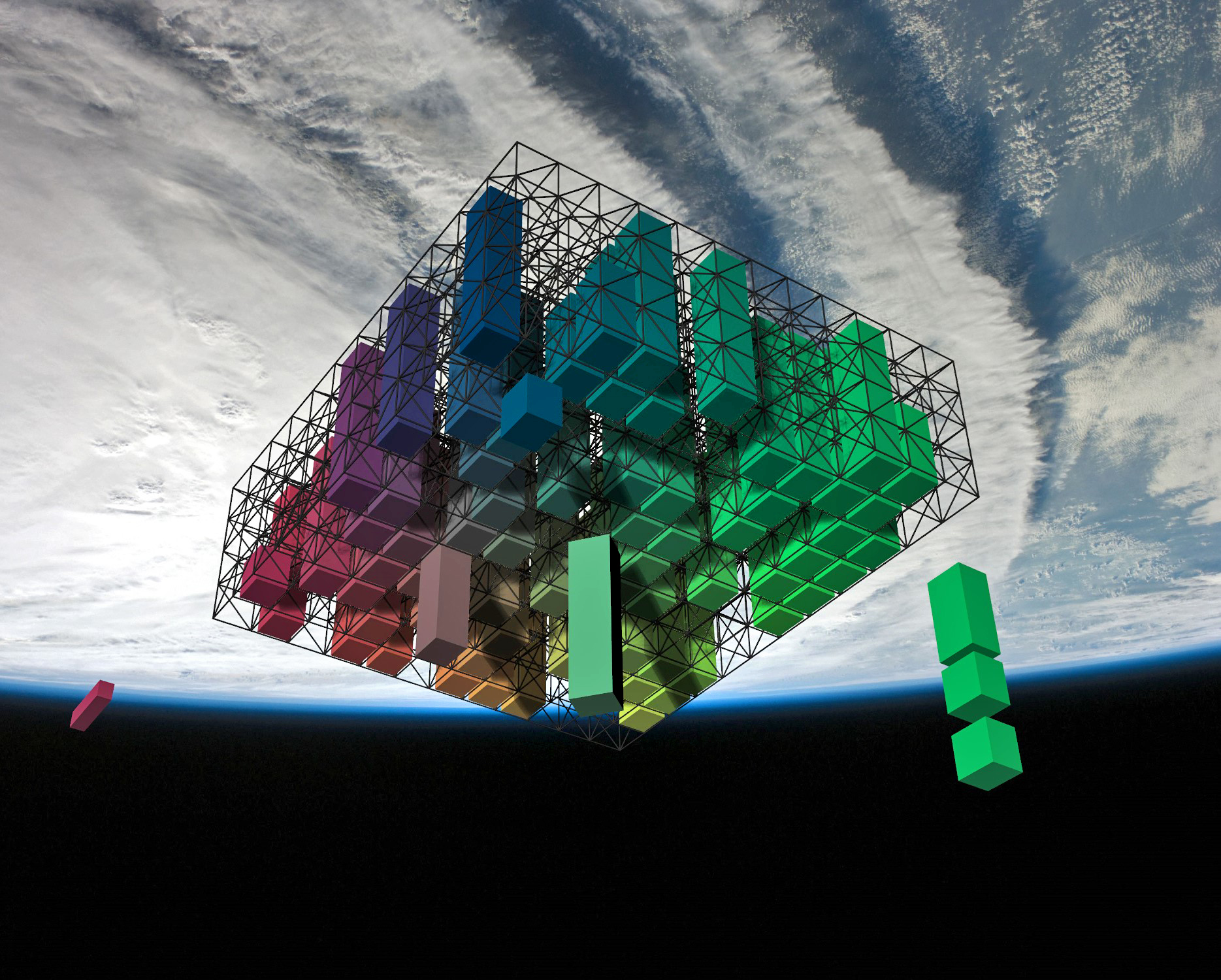
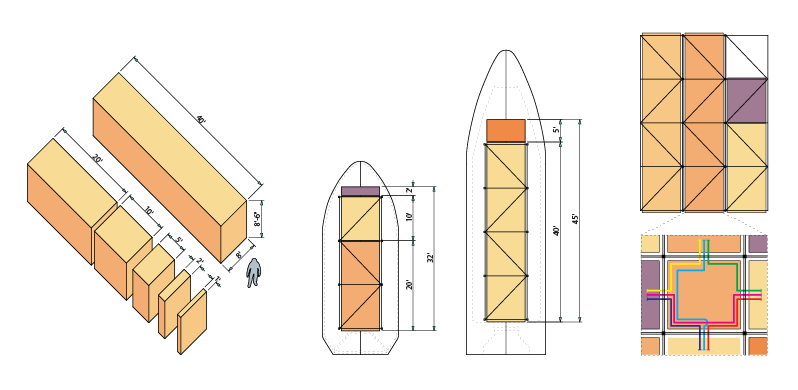
The module size is derived from the standard shipping container so that it can leverage the Global Intermodal Freight Transport System (GIFTS) – an infrastructure providing total and affordable access to all of Earth’s biological communities. ECOTOPE offers a use case for amending rockets to the list of existing transport modes (e.g. trains, boats, trucks, planes) to expand a planetary infrastructure into an orbital one and in doing so exploit modularity to study ecological dynamics.
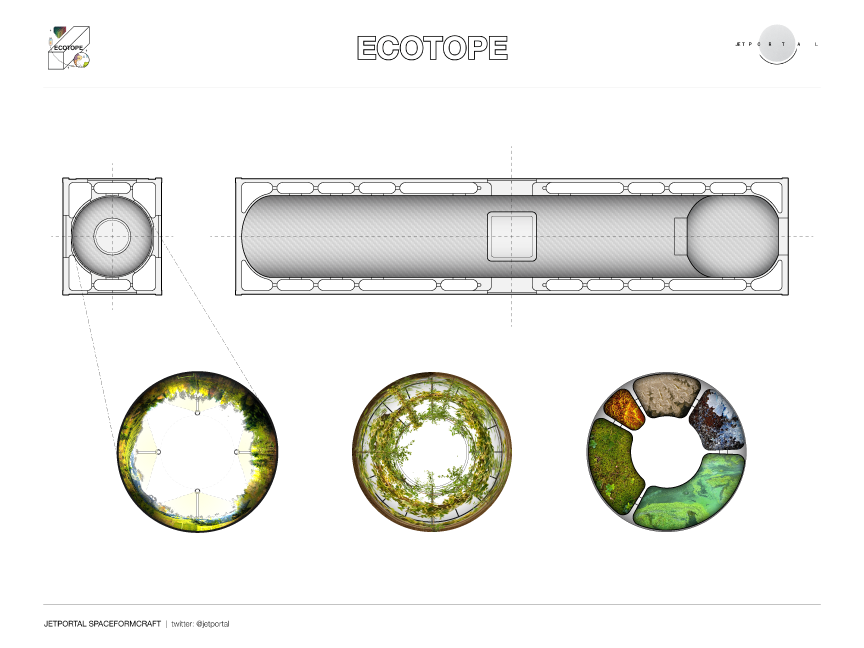
In landscape and spatial ecology, the term ecotope refers to the smallest spatial unit or component of an ecological landscape. Ecotopes are considered relatively homogeneous within themselves but can form heterogeneous and complex landscapes when connected, producing emergent behavior such as gene flow, population dynamics, seed dispersal, storage effects, and pathogen virulence. Ecotope would allow researchers to upscale modern ecological experiments so that these large-scale phenomena can be studied for the first time under controlled conditions.
Ecotope will offer the first studies of ecosystems and heterogeneous landscapes unrestricted by scale or geographical origin and Space access to our home biosphere, on which Space settlement will remain dependent for decades to come. In contrast to projects like Biosphere 2 that staged holistic ecological research in an idealized mono-volume replica of Earth, Ecotope leverages existing infrastructure to provide the means to study and select functional ecosystem traits through an evolvable network of smaller architectures.
Project team
Jeffrey Montes (PI/PD)
Nicholas Palermo (Co-I)
Nicholas Palermo (Co-I)
References
Chesson, P. (1998). Making sense of spatial models in ecology. Modeling spatiotemporal dynamics in ecology, 151-166.
Doak, D. F., Bigger, D., Harding, E. K., Marvier, M. A., O'malley, R. E., & Thomson, D. (1998). The statistical inevitability of stability‐diversity relationships in community ecology. The American Naturalist, 151(3), 264-276.
Ellis E. C., H. Wang, H. Xiao, K. Peng, X. P. Liu, S. C. Li, H. Ouyang, X. Cheng, and L. Z. Yang. 2006. Measuring long-term ecological changes in densely populated landscapes using current and historical high resolution imagery. Remote Sensing of Environment 100(4):457-473.
Ellis, Erle. (2008). Ecotope. Encyclopedia of Earth.
Holling, C. S. (1973). Resilience and stability of ecological systems. Annual review of ecology and systematics, 1-23.
MacArthur, R. (1955). Fluctuations of animal populations and a measure of community stability. ecology, 36(3), 533-536.
McRae, B. H., Dickson, B. G., Keitt, T. H., & Shah, V. B. (2008). Using circuit theory to model connectivity in ecology, evolution, and conservation. Ecology, 89(10), 2712-2724.
Snyder, R. E., & Chesson, P. (2003). Local dispersal can facilitate coexistence in the presence of permanent spatial heterogeneity. Ecology Letters, 6(4), 301-309.
Voris, P. V., O'Neill, R. V., Emanuel, W. R., & Shugart, H. H. (1980). Functional complexity and ecosystem stability. Ecology, 61(6), 1352-1360.
Warner, R. R., & Chesson, P. L. (1985). Coexistence mediated by recruitment fluctuations: a field guide to the storage effect. American Naturalist, 769-787.
Wilson, David Sloan. (1997)."Biological communities as functionally organized units." Ecology 78.7, 2018-2024.
Doak, D. F., Bigger, D., Harding, E. K., Marvier, M. A., O'malley, R. E., & Thomson, D. (1998). The statistical inevitability of stability‐diversity relationships in community ecology. The American Naturalist, 151(3), 264-276.
Ellis E. C., H. Wang, H. Xiao, K. Peng, X. P. Liu, S. C. Li, H. Ouyang, X. Cheng, and L. Z. Yang. 2006. Measuring long-term ecological changes in densely populated landscapes using current and historical high resolution imagery. Remote Sensing of Environment 100(4):457-473.
Ellis, Erle. (2008). Ecotope. Encyclopedia of Earth.
Holling, C. S. (1973). Resilience and stability of ecological systems. Annual review of ecology and systematics, 1-23.
MacArthur, R. (1955). Fluctuations of animal populations and a measure of community stability. ecology, 36(3), 533-536.
McRae, B. H., Dickson, B. G., Keitt, T. H., & Shah, V. B. (2008). Using circuit theory to model connectivity in ecology, evolution, and conservation. Ecology, 89(10), 2712-2724.
Snyder, R. E., & Chesson, P. (2003). Local dispersal can facilitate coexistence in the presence of permanent spatial heterogeneity. Ecology Letters, 6(4), 301-309.
Voris, P. V., O'Neill, R. V., Emanuel, W. R., & Shugart, H. H. (1980). Functional complexity and ecosystem stability. Ecology, 61(6), 1352-1360.
Warner, R. R., & Chesson, P. L. (1985). Coexistence mediated by recruitment fluctuations: a field guide to the storage effect. American Naturalist, 769-787.
Wilson, David Sloan. (1997)."Biological communities as functionally organized units." Ecology 78.7, 2018-2024.